
Galectin-3: The protein paving new treatment avenues
pharmafile | November 1, 2018 | Feature | Business Services, Manufacturing and Production, Medical Communications, Research and Development, Sales and Marketing | Galectin-3, Galectins, breast cancer, diabetes, heart disease, pharma
Matthew Blakeley, LADI-III Beamline Scientist at Institut Laue-Langevin (ILL) and Derek Logan, Associate Professor in Structural Biology at Lund University, discuss galectin-3 and its link to diabetes, heart disease and breast cancer, and how it could lead us to new therapies.
Can you provide a bit of background on galectins and why our understanding of them is important in the discovery/design of new treatments?
Galectins are a large and ancient class of sugar-binding proteins characterised by their binding to galactoside, a carbohydrate found on proteins on the surface of many cells. They take part in a vast number of physiological functions, including the regulation of inflammation, pathways in the immune system, and communication between cells.
We are interested in galectins because of the hugely important role that they play throughout the body, their distribution throughout almost all cells and tissues, and their subsequent implication in a range of diseases involving the functions they regulate. There is evidence that galectins are involved in various cancers, cardiovascular disease, HIV and autoimmune disease; all devastating and widespread chronic conditions.
As the galectins carry out their functions through the critical interaction with sugars, enhancing our understanding of the exact mechanisms in this binding process could aid us in the development of inhibitors. By blocking the protein’s actions, the development of certain diseases could be limited.
Our particular interest is in galectin-3. This galectin has been strongly linked to diseases including diabetes, heart disease, and breast cancer, which is globally the most common cancer in women, with 1.7 million new cases diagnosed in 2012 alone. These associations are thought to be a result of its role in cell-to-cell adhesion, cell growth, and cell differentiation, as its upregulation is linked to increased tumorigenesis in cancer, and fibrosis in heart, lung, and renal disease.
Please can you outline the new findings on the nature of galectin-3 and its binding interactions? What exactly was discovered and why is it significant?
Studying the exact binding mechanisms for galectin-3 has been difficult. We know the interaction largely involves hydrogen bonds and water molecules but ascertaining the exact position of hydrogen atoms using analytical tools such as X-rays is challenging, due to the weak scattering of hydrogen with X-rays. Even with the most high-resolution X-ray crystallography, it has been mostly guesswork, as only about half of the most ordered hydrogen atoms could be explicitly identified.
Our new findings came as a result of using neutron crystallography in combination with X-rays to analyse the exact binding mechanisms. At the Institut Laue-Langevin (ILL) in Grenoble, France, we house the world’s most intense neutron beam, and a range of world-leading neutron crystallography tools for investigating atomic structure. Neutron crystallography is an ideal technique for revealing the precise positions of hydrogen atoms, and subsequently, the geometry of hydrogen bonds. Unlike X-rays, hydrogen atoms scatter neutrons to approximately the same magnitude as the other common elements in a protein. X-ray crystallography enabled us to only infer the position of hydrogen atoms based on the position on heavier atoms, whereas neutron crystallography gives us the full picture.
Using the LADI-III beamline, we were able to reveal the exact hydrogen bonding networks for the C-terminal carbohydrate recognition domain of galectin-3 (galectin-3C) which, combined with X-ray crystallography data, gives us a much better understanding of the interaction of galectin-3 with its binding target. In addition, through the elucidation of the structure of sugar-free galectin-3C, we have been able to observe the positions and orientation of water molecules in the binding site. By comparing the sugar-free and sugar-bound structures, we can see how the interaction is altered upon binding, and understand the role of water molecules in the process. These findings may open the doors to more efficient, targeted drug development to find an inhibitor of this process, as we have significantly more detail on the atomic factors at play.
How was the breakthrough achieved? How did collaboration help to bring the research to fruition?
The LADI-III beamline at the ILL is a neutron diffractometer specifically designed for use in biological research – it is ideally suited to locating protons and deuterons, making it perfect for looking at enzyme mechanisms or drug-ligand binding interactions.
In addition, we needed X-ray crystallography to be carried out to a very high resolution, giving a clear and accurate picture of all of the heavier atoms involved in the binding process. Amongst the most powerful X-ray beamlines are the instruments at the European Synchrotron Radiation Facility (ESRF), which shares a site in Grenoble with the ILL. The ESRF and ILL were the location for much of the study, which was also a collaboration with Lund University (Sweden, including the MAX IV Laboratory), Oak Ridge National Laboratory (USA) and Heinz Maier-Leibnitz Zentrum (Germany). We managed to make the breakthrough thanks to the powerful complementary techniques of neutron and X-ray crystallography, which together form a potent analytical tool for characterising interactions between proteins and their ligands.
What implications does this have for drug development? Does this present a new target for R&D efforts? Can you provide some examples of how this may influence or change the drug discovery process within relevant diseases?
To develop an effective inhibitory drug, a thorough and complete picture of the target binding process is essential.
Our findings from neutron crystallography can greatly aid drug discovery processes that use molecular simulations and computational models of ligand binding to identify candidates. The calculations that form the foundations of these models depend heavily on the knowledge of the exact atomic bonding network, which is impacted by the location of hydrogen atoms. By precisely locating these atoms in galectin-3C experimentally, we can provide an improved foundation for the development of novel inhibitors through computational methods.
Successful inhibitors of galectin-3 have been developed before, but have largely been based on molecules that closely resemble natural ligands of the protein. The characterisation of the hydrogen bonding networks we have achieved will also help refine and improve these existing drugs.
Heart disease remains the biggest threat to health worldwide while breast cancer remains the most common cancer for women around the world. These have been cited as areas where the research may have the biggest impact – how exactly will it help?
The exact role that galectin-3 potentially plays in the development and spread of malignant cells differs depending on the type of cancer. In breast cancer, there is evidence to suggest that it enhances the adherence of breast cancer cells to others in the human body, critically including the extracellular network of cells that connects different tissues in the body. This suggests that it may have a role in metastasis, facilitating the spread of cancerous cells throughout the body. An accurate picture of the mechanisms by which this protein enables the dissemination of malignant cells could be targeted by novel therapeutics, and so characterisation of the binding process using neutrons opens the doors to this research.
Galectin-3 plays an important role in the regulation of fibrosis in the liver, kidney, and the heart. Cardiac fibrosis is a key contributing factor in the development and progression of heart failure, and overexpression of galectin-3 in conjunction with advanced cardiovascular disease strongly suggests that by preventing its binding mechanisms, the resulting reduction in fibrosis could make the inhibitor a powerful therapeutic.
Breast cancer treatment has made great strides since the advent of immuno-oncology therapies on the market. While they have proven effective, what could this new research mean for refining treatment of the disease?
Immuno-oncology drugs are antibodies, which means their production is very expensive, a cost that is passed onto the patient. Furthermore, they typically have to be injected. Small-molecule drugs such as those that are being developed against galectin-3 are cheaper and also preferable in that they can be taken orally.
Related Content
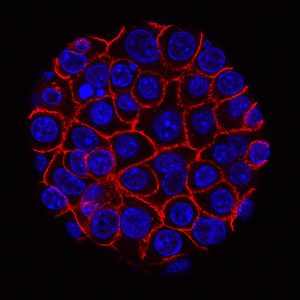
FDA accepts BLA for AstraZeneca and Daiichi Sankyo’s datopotamab deruxtecan for breast cancer treatment
AstraZeneca and Daiichi Sankyo have announced that their Biologics License Application (BLA) for datopotamab deruxtecan …

CHMP recommends Novo Nordisk’s Awiqli for diabetes treatment
Novo Nordisk has announced that the European Medicines Agency’s (EMA) Committee for Medicinal Products for …

Roche shares data on novel solution for continuous glucose monitoring
Roche has shared the latest data on its novel solution for continuous glucose monitoring (CGM), …